EV Battery Degradation: Key Research Insights & Tips
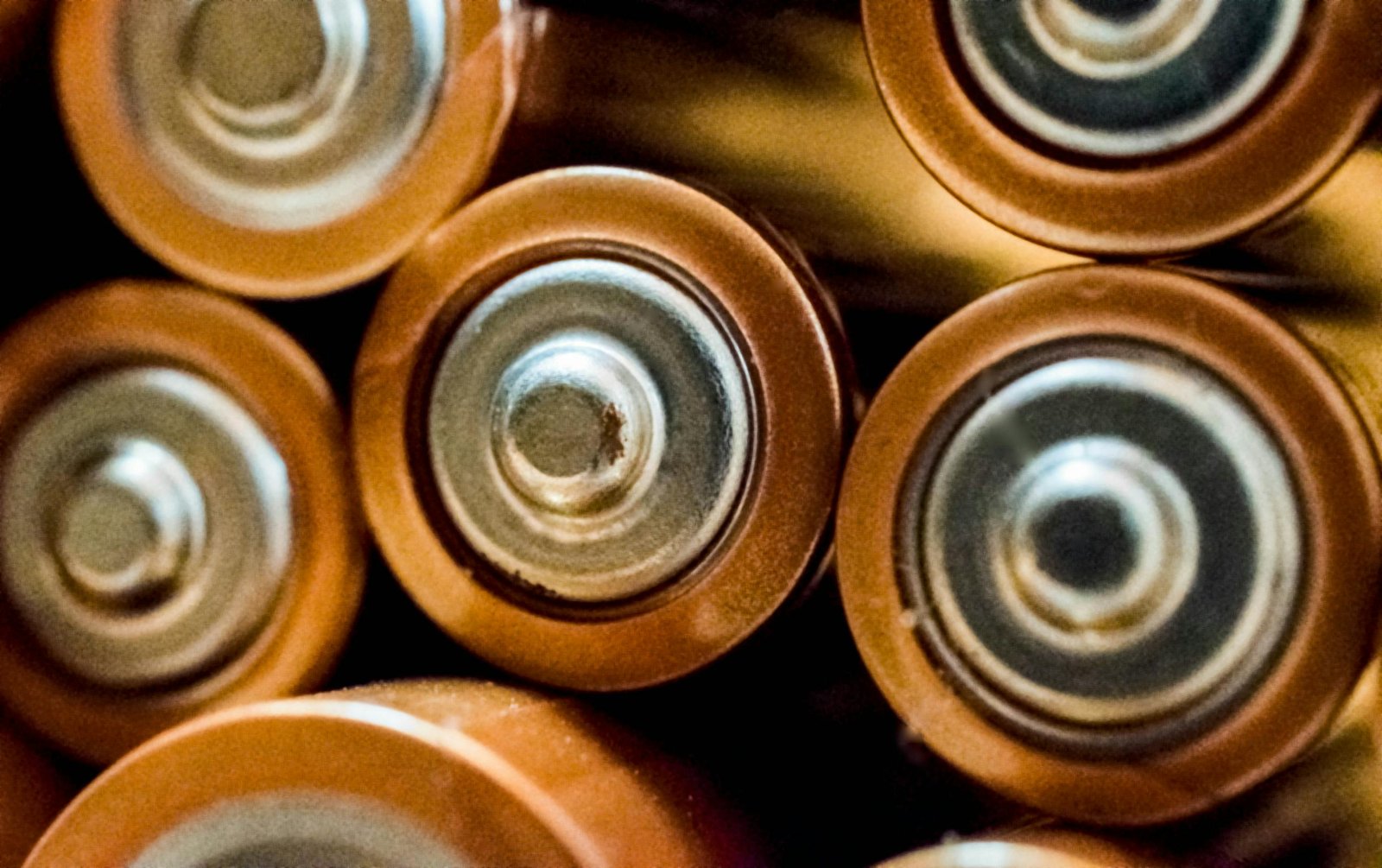
Electric vehicles (EVs) have gained immense popularity due to their eco-friendliness, performance, and lower running costs. However, a critical challenge that EV owners face is battery degradation. The battery is the heart of an EV, directly influencing its range, performance, and lifespan. Understanding the scientific basis of battery degradation can help EV owners make informed choices to extend the life of their vehicle’s battery.
This comprehensive blog will delve into the key factors influencing battery degradation, highlight research findings, and offer practical tips to minimize degradation. It will also provide a focused discussion on different battery chemistries, including Lithium Iron Phosphate (LFP) batteries. This article is based on the research findings from the IEEE review article “Battery Degradation in Electric and Hybrid Electric Vehicles: A Survey Study” which provides an in-depth analysis of battery degradation mechanisms and mitigation strategies.
What is Battery Degradation?

Battery degradation is the gradual loss of capacity and performance of a battery over time, mainly due to the chemical reactions that occur inside the battery cells. These reactions can be influenced by various factors such as temperature, charging habits, depth of discharge (DoD), and cycle life.
The research findings from the IEEE indicate that battery degradation in EVs is a complex phenomenon influenced by a range of factors that interact in different ways to affect the battery’s overall lifespan and performance.
Factor 1: Temperature Effects on EV Batteries
Temperature is a critical factor influencing battery degradation. According to the survey study, high temperatures accelerate the rate of chemical reactions within the battery cells, leading to faster degradation of key components like the electrolyte and electrodes. Conversely, extremely low temperatures can increase internal resistance, reducing the battery’s ability to deliver power efficiently.
Research shows that at high temperatures, the electrolyte inside the battery breaks down faster, leading to capacity loss. For example, studies have found that at temperatures above 30°C (86°F), the rate of electrolyte decomposition doubles with every 10°C (18°F) increase, significantly shortening the battery’s lifespan .
Elevated temperatures can cause lithium plating on the anode, which reduces battery capacity and can potentially cause short circuits. It also accelerates the growth of the Solid Electrolyte Interphase (SEI) layer, a protective barrier that forms on the anode and gradually thickens, leading to increased internal resistance and reduced capacity .
In extreme cases, high temperatures can lead to thermal runaway, where the battery heats up uncontrollably, causing rapid degradation or even safety hazards like fires.
Conversely, low temperatures can reduce charging rates and capacity. Research found that charging at very low temperatures can result in incomplete lithium ion insertion and reduced overall battery capacity.
Practical Recommendations to Manage Temperature
Whenever possible, park your EV in a garage or shaded area to avoid temperature extremes.
Many EVs offer a preconditioning feature that warms or cools the battery before use, which can help mitigate the effects of extreme temperatures.
Try to keep your vehicle within moderate temperature ranges (20-25°C or 68-77°F) to reduce temperature-induced degradation.
Factor 2: Charging Speed on EV Battery Health
The research highlights that charging speed significantly impacts battery life. Frequent use of fast chargers, which operate at high power levels (often above 50 kW), can generate excessive heat, accelerating the chemical degradation processes within the battery. In contrast, slow charging (using Level 1 or Level 2 chargers at lower power levels) allows the battery to cool between charging cycles, reducing overall wear and tear .
High charging currents cause rapid heating of the battery cells, which accelerates degradation. For instance, the study found that fast charging cycles increased the battery’s internal temperature by up to 15°C, which can significantly shorten battery life if used frequently.
High charging rates also increase the risk of lithium plating on the anode, which permanently reduces capacity and can lead to battery failure. This risk is especially pronounced at low temperatures, where lithium ions move more slowly.
The charging current is typically measured in terms of C-rate, where 1C equals a charging current that would fully charge the battery in one hour. A study on different charging rates revealed that batteries charged at 10C experienced a significant reduction in lifecycle count compared to those charged at 1.25C. Specifically, batteries charged at 1.25C had a lifecycle count of 2950 cycles, while those charged at 10C had only 414 cycles.
Practical Recommendations for Charging
Use fast chargers sparingly, such as during long trips or emergencies, to avoid excessive heat buildup.
For daily use, keep the battery state of charge (SoC) between 20% and 80% to minimize stress on the battery cells.
Take advantage of off-peak hours to charge the vehicle when ambient temperatures are lower and electricity rates are cheaper.
Factor 3: Depth of Discharge (DoD) Impact on EV Battery Health
Depth of Discharge (DoD) is another critical factor affecting battery degradation. DoD refers to the percentage of a battery’s capacity that has been discharged relative to its total capacity. Research findings indicate that a higher DoD (e.g., regularly discharging the battery to 10% or lower) can significantly shorten the battery’s lifespan.
The study demonstrates that batteries discharged to lower states of charge (e.g., 10% or less) have up to 90% fewer charge cycles compared to those maintained at a shallower DoD (e.g., 80-90%) .
For instance, at a constant temperature of 15°C, a battery discharged by 90% (from 100% to 10% SoC) had a cycle count of 2,144, whereas a battery discharged by only 10% had a cycle count of 18,067.
Recommendations to Manage Depth of Discharge
Try to keep the battery level above 20% most of the time to reduce the stress on the battery cells.
Regularly charging the battery up to 80% rather than 100% can help minimize the impact of DoD on battery degradation.
Factor 4: Cycle Life and Aging
Battery cycle life is defined as the number of complete charge and discharge cycles a battery can undergo before its capacity falls below a usable level (usually 70-80% of its original capacity). Research indicates that each cycle contributes to battery aging and degradation, but factors like temperature, DoD, and charging patterns significantly influence the rate of degradation.
High rates of charging and discharging increase the stress on the battery cells, reducing the number of cycles it can withstand. For example, high discharge rates can cause temperatures to spike and accelerate SEI layer growth, leading to increased resistance and capacity loss .
The study found that at a constant discharge rate of C/2, batteries discharged at higher temperatures had significantly fewer cycles compared to those discharged at room temperature. This indicates that maintaining optimal temperatures can extend cycle life by reducing degradation.
Recommendations to Extend Cycle Life
Use the vehicle in regular, predictable driving patterns to stabilize cycle life and minimize extreme charge or discharge rates.
If your EV supports V2G operations, limit deep discharge cycles to reduce wear on the battery.
LFP vs. NMC Battery Degradation
Research shows that lithium iron phosphate (LFP) batteries are more stable at high temperatures compared to nickel manganese cobalt (NMC) batteries. LFP batteries are less prone to thermal runaway, a dangerous situation where excessive heat causes uncontrollable reactions, making them safer under high-temperature conditions. Although both types of batteries degrade with heat, LFP batteries experience less severe effects. For example, NMC batteries lose capacity and develop internal resistance when temperatures exceed 25°C, whereas LFP batteries maintain stable performance over a wider range. NMC batteries are also more vulnerable to cold temperatures, which can cause rapid degradation, while LFP batteries remain more resistant.
When it comes to depth of discharge (DOD), LFP batteries perform better than NMC batteries under high DOD conditions. Research indicates that LFP cells can withstand frequent deep discharges (90% or higher) without significantly reducing their cycle life. This resilience is due to the structural stability of the lithium iron phosphate material, which remains intact under heavy use. In contrast, NMC batteries degrade faster at high DODs, as their materials are more prone to structural changes and capacity loss. This makes LFP batteries better suited for applications that require regular, deep cycling.
LFP batteries also handle high discharge currents more effectively, which is essential for electric vehicles that require rapid acceleration or frequent use of high-power modes. While both LFP and NMC batteries can degrade at high discharge rates, LFP batteries are less susceptible to rapid performance loss. Research shows that LFP batteries can handle high current loads without significant degradation, whereas NMC batteries, despite their higher energy density, may degrade faster due to increased internal resistance and material breakdown.
LFP batteries generally have a longer cycle life than NMC batteries. The stable chemistry of LFP allows these batteries to endure more charge and discharge cycles before reaching the end of their useful life. Studies have shown that LFP batteries can complete a high number of cycles, even under stressful conditions like high temperatures or deep discharges. In contrast, NMC batteries, while providing higher energy density and longer range, tend to degrade faster and reach their end of life sooner, particularly when exposed to extreme temperatures or deep discharges.
How a Battery Management System (BMS) Reduces Degradation
For EV users and potential adopters, understanding the role of the BMS is vital. It not only protects the battery from the factors that cause degradation but also enhances its performance and safety. The BMS is like the “brain” of the battery, constantly monitoring and adjusting parameters to maintain a balance between performance, safety, and longevity. Knowing this can help you make more informed decisions about how you drive, charge, and maintain your EV, ultimately maximizing its life and value.
The BMS is equipped with thermal sensors that monitor the temperature of each battery cell in real time. It can adjust cooling systems, such as fans or liquid coolants, to maintain an optimal temperature range. For example, if the temperature is too high, the BMS may activate cooling systems to dissipate heat, preventing overheating and the associated rapid degradation. If the temperature is too low, the BMS can preheat the battery to avoid lithium plating and other low-temperature effects.
Studies have shown that keeping the battery within a moderate temperature range can significantly extend its life. For instance, operating at temperatures above 45°C can accelerate the growth of the SEI layer on the negative electrode, increasing internal resistance and reducing capacity. A BMS mitigates this risk by actively controlling the temperature, thus slowing down degradation.
A BMS monitors the discharge rate of the battery to prevent excessive current from damaging the cells. High discharge currents can cause excessive heating and stress on the battery materials, leading to faster degradation. By regulating the maximum allowable discharge current, the BMS reduces the risk of overheating and thermal runaway, which is a condition where the battery temperature continues to rise uncontrollably.
During charging, the BMS controls the charging current to minimize stress on the battery cells. High charging currents, especially in fast charging scenarios, can lead to increased heat generation and rapid degradation. The BMS can adjust the current flow to keep it within safe limits, thereby reducing thermal and mechanical stress. This is particularly important at the beginning and end of the charging process when the battery is most vulnerable to damage.
Lack of Real-World Data
One significant research gap is the lack of comprehensive real-world battery data, which hinders the development of accurate degradation models. Current research often relies on laboratory data from limited test conditions, which do not fully represent the diverse and uncontrolled environments in which electric vehicle (EV) batteries operate. Most studies use data from hybrid electric vehicles (HEVs) or specific test scenarios, leaving a gap in understanding how batteries perform under various real-world conditions. To address this, it is crucial to gather extensive field data from actual EVs, including diverse driving patterns and environmental factors, to create more robust and representative degradation models.
Another critical gap is the need for advanced, self-learning algorithms capable of real-time degradation prediction. Existing models often use static or offline approaches that do not adapt well to changing conditions or new data. To improve prediction accuracy, there is a need for algorithms that can dynamically update and learn from real-time data, accounting for uncertainties and evolving battery conditions. Developing scalable, hybrid algorithms that combine data-driven and model-based methods could enhance the precision of degradation forecasts and extend battery life by minimizing deterioration while optimizing vehicle performance.
The Electric Viking reported that in real-world scenarios, a sample of approximately 30 Tesla Model 3 vehicles averaged around 480,000 kilometres with only 10% battery degradation on average. This data provides buyers with confidence that a Tesla Model 3 battery can last throughout the vehicle’s entire lifespan under typical driving conditions.
Many Tesla Model 3 and Model Y owners on Twitter have reported that their batteries have degraded by 8% even after driving over 200,000 km. This aligns with the real-world battery degradation data that Tesla shared on Twitter.
“Our batteries are designed to outlast the life of the vehicle—after 200k miles (or 321k km), Model 3 & Y retain 85% of their original capacity on average”
TechWheel’s Take
In reality, modern EV batteries are designed to outlast the vehicle itself. While factors like weather, driving style, and charging patterns significantly impact battery health, as demonstrated in research, EV manufacturers take these factors into account and develop highly intelligent Battery Management Systems (BMS). These systems ensure that the battery is protected even in stressful situations. Additionally, ongoing improvements in battery technology and BMS design are leading to less degradation over time. I hope this article provides well-documented insights backed by scientific research.
2 responses to “EV Battery Degradation: Key Research Insights & Tips”
-
I really like reading through a post that can make men and women think. Also, thank you for allowing me to comment!
-
I just like the helpful information you provide in your articles
Leave a Reply